How is cailabs contributing to improving communication with satellites?
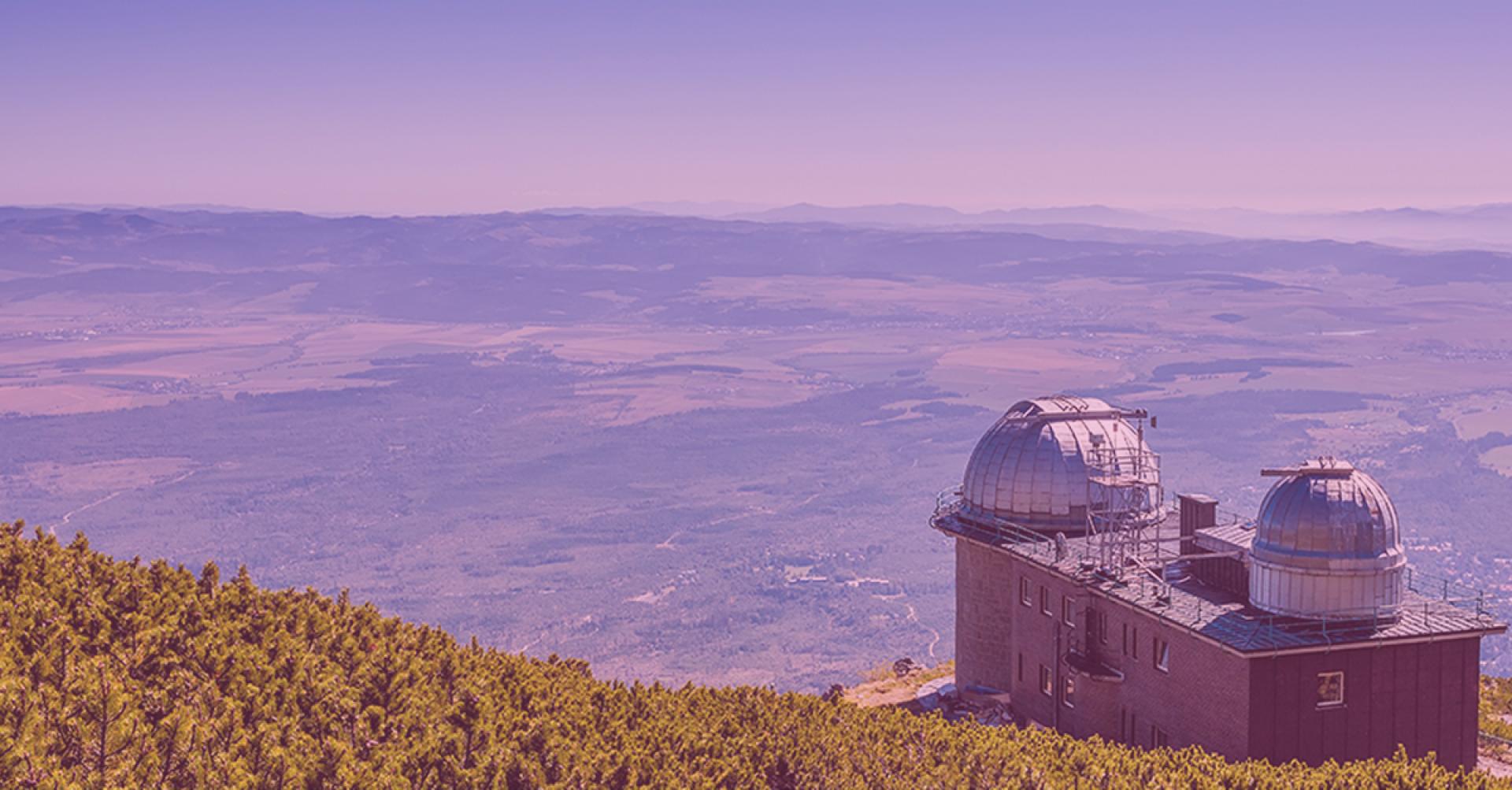
Satellite constellations enable the deployment of high-throughput communication networks in free space to complement fiber-optic networks. Like these networks, optical technologies are the only means to ensure the throughputs required. In this article, we show how Cailabs technology can improve optical telecommunication links with satellites.
Space, the next frontier for communication networks
It is especially evident during the current health crisis – telecommunications networks have become a critical element to ensure that society’s communication needs are met. They allow us to chat with friends and family, to consult doctors, to work, to contact local or large retail stores, to read the news, to visit museums, to listen to music and to plan our trips for the holidays. All areas of our society, from health to defense, from culture to food, are now digitized and rely on telecommunications networks.
In light of this, two questions arise. Firstly, how can we guarantee a network connection for everyone when 50% of the world’s inhabitants still do not have access to it? Secondly, how can we ensure that the system is resilient, i.e. that it is capable of overcoming changes in its environment? According to the ADEME, an item of digital data travels an average of 15,000 km, in other words, well beyond national borders. So, how can we ensure access to network infrastructures in the event of natural disasters, malicious acts or even conflict? It is often said that the Internet is globally robust but locally vulnerable.
One of the solutions to address these two challenges, which is emerging thanks to New Space and easier access to space, is to use satellite constellations to deploy high-throughput networks to complement fiber-optic networks. However, to guarantee the same throughput as optical fiber, optical technologies must be used for these networks too. We worked with the German space center, DLR, to show how Cailabs technology can improve these communication networks.
Comparison of TILBA® vs. Adaptive Optics using an experimental demonstration of a GEO link
A laboratory of international expertise
DLR (Deutsches Zentrum für Luft- und Raumfahrt) is the German space research center. The DLR’s Institute of Communications and Navigation is working on the design of communication networks, and specifically on laser communication technologies. In particular, the Advanced Optical Technologies Group holds the throughput record for optical links in free space, achieving transmissions of up to 13.65 Tbit/s in the case of feeder-links (uplinks to a satellite) in real-life conditions. DLR is thus one of the experts in optical telecommunications links.
Using standard telecommunication components: the challenge of laser communications.
To create a high-throughput link, it is essential to use standard telecom equipment such as coherent transceivers and amplifiers, which are available mainly on single-mode optical fibers. These components, which benefit from the technological maturity of fiber-optic networks, are the only ones that meet the performance requirements in terms of speed, noise and sensitivity while remaining economically competitive.
Unfortunately, the phenomenon of atmospheric turbulence deteriorates coupling efficiency in the optical fiber by modifying the profile of the light wavefront. The result is a degradation of the optical link, i.e. an increase in the number of errors and interruptions of the link. In order to establish high-throughput links with GEO or LEO satellites, therefore, an atmospheric turbulence compensation system is required to efficiently couple the disrupted incident wavefront into a standard optical fiber.
DLR researchers have extensive expertise in uplinks and, more generally, in optical transmissions in free space. They are also interested in downlinks and systems to compensate for turbulence at reception.
In the article co-published by DLR and Cailabs, two technologies are compared: a standard adaptive optics system and the first version of Cailabs’ TILBA®-ATMO, without optical recombination. Output scintillation at the receiver is evaluated in both cases to determine the efficiency of turbulence compensation.
Two technologies with the same functionality
Adaptive optics consists of reshaping the wavefront using a deformable mirror. Figure 1 shows a typical adaptive optics system. The incident signal is sent to the deformable mirror which corrects wavefront distortion. A fraction of the corrected signal is sent to a wavefront sensor to characterize its quality in real time. Finally, the other fraction of light is sent through the optical fiber to the telecommunication receiver. For this study, a wide-field photodiode was also used to measure the total intensity fluctuation of the wavefront in the focal plane.
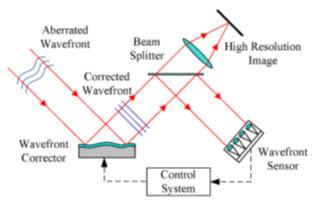
Although its function is similar, the approach of the TILBA®-ATMO is different. Its objective is not to actively compensate for wavefront distortion, but rather to collect all the light and passively convert it into usable modes. A light wave can be decomposed into modes. In this case, we have chosen Hermite-Gauss modes. The Mutli-Plane Light Conversion technology (MPLC) on which the TILBA®-ATMO is based, then collects these various disruptive modes and demultiplexes them into as many single-mode fibers. The dynamics of turbulence still exist, but they are reflected in variations in phase and intensity of the modes, which themselves are fixed. After demultiplexing, these variations result in changes in phase and intensity of the respective signals, but in the single-mode fibers.
This passive approach makes it possible to compensate for the phase shift in the fibers themselves rather than with mechanical elements, which are intrinsically limited in speed. Finally, it should be noted that if adaptive optics also use phase mode , such as Zernike polynomials in turbulence analysis. MPLC works on the complex field, i.e. both the phase and the amplitude.
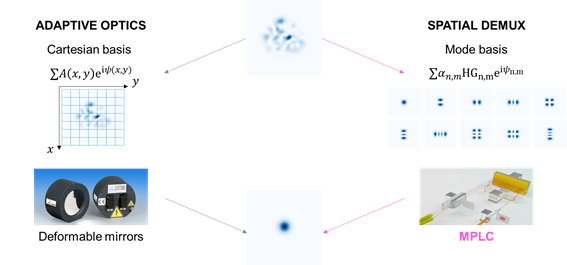
Experimental demonstration of a GEO downlink signal
The DLR experiment took place between the DLR station in Weilhem, acting as the ground station, and a terminal, representing the satellite, located at the weather station in Hohenpeißenberg. The two sites are 10.45 km apart with an inclination of about 1.8°. This site, already used by the DLR for several test campaigns, has turbulence and scintillation characteristics that are representative of a GEO-ground link, which make it particularly suitable for the study of communication systems.
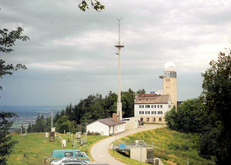
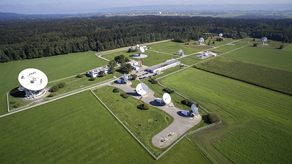
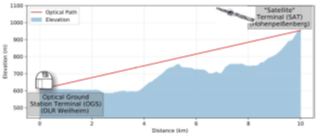
The test bench is shown in Figure 4. Once collected by a 10 cm diameter telescope, a tip/tilt mirror stabilizes the beam pointing direction. The signal is then separated into two equal fractions. Part of the signal is sent to the MPLC while the other part is sent to the adaptive optics unit.
TILBA®-ATMO: The MPLC input is a few-mode fiber by Prysmian with a core diameter of 25 µm guiding 10 modes. The modes of the fiber are demultiplexed into 10 single-mode fibers. This first version of TILBA has no recombination device. Each single-mode fiber is therefore connected to a gain-adjustable PIN photodiode from Thorlabs. The analog signal obtained on each photodiode is oversampled and then digitally summed to obtain a single output corresponding to the signal compensated by the TILBA.
Adaptive optics: In parallel, the adaptive optics unit comprises a deformable mirror with 97 actuators which couples the beam directly into a single-mode fiber connected to a photodiode. Part of the signal is sent to a Shack-Hartmann wavefront analyzer which enables the control of the deformable mirror, and the estimation of the Fried parameter. A wide-angle camera is used to measure the signal intensity in the focal plane.
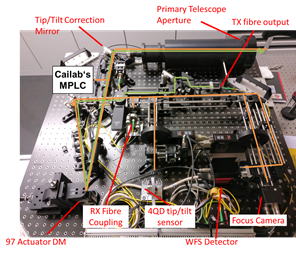
Similar performance to adaptive optics, and even better in high turbulence conditions.
Adaptive optics and TILBA®-ATMO were compared in three turbulence configurations corresponding to three types of scintillation in the focal plane σ²: low for σ²< 0.1, medium for 0.1 < σ² < 0.3 and high for 0.3 < σ². In each case, the scintillation in the receiver plane after correction was measured. The lower the scintillation, the more effective the correction and therefore the better the performance.
The results obtained are extremely promising. They show that the performances are equivalent for TILBA®-ATMO and the adaptive optics system in the case of low and medium turbulence. However, in cases of high turbulence, which experimentally correspond to situations where the error and link interruption rate is at its highest, TILBA®-ATMO achieves better performance than adaptive optics. The dynamic range of the wavefront sensor is pushed to the limit whereas TILBA®-ATMO, being a passive component, is not affected by this type of limitation.
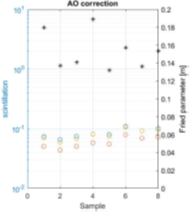
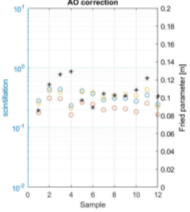
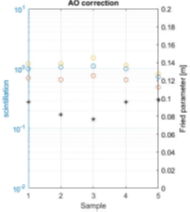
Figure 5: Comparison of scintillation in cases of low (left), medium (middle) and high turbulence between adaptive optics (in yellow) and TILBA®-ATMO (in blue) in the receiver plane. The weaker the scintillation, the more effective the correction. The red circles represent the scintillation in the focal plane, which can result in a perfect correction. The black stars represent Fried’s parameter.
What’s next for TILBA®-ATMO?
The first tests carried out by NEC starting in 2016 were already extremely promising. However, while the Japanese researchers were studying the link from a telecommunications point of view, the experiments took place in the laboratory with emulated turbulence. The tests presented here are the first to be carried out in real-life conditions with turbulence representative of a GEO link. Above all, this experiment is the first to perform a real-time comparison of the performance of an adaptive optics system to that of an MPLC. These measurements show the power of the TILBA®-ATMO approach.
There are, of course, a number of issues inherent in test systems which deserve further consideration. For example, future campaigns will study the impact of the recombination device, provinding a single output on single-mode fiber, which is essential for a complete comparison with an AO system, providing a single output on single-mode fiber. Several telescope sizes can also be considered, as well as different numbers of modes for the MPLC. Finally, to validate the TILBA®-ATMO approach, Cailabs will test its components on operational links with satellites.
However, the results of this paper already confirm the relevance of a passive modal approach to provide an off-the-shelf atmospheric turbulence compensation system.
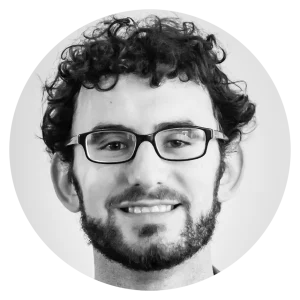
By David Allioux
David Allioux, who holds a Ph.D. in optics from the University of Lyon, joined Cailabs in 2018. As a project and product manager, he is in charge of the development and integration of telecommunication R&D solutions. He also brings his expertise to develop and commercialize the laser communication product line.