How to reach 10 pb/s in single optical fiber?
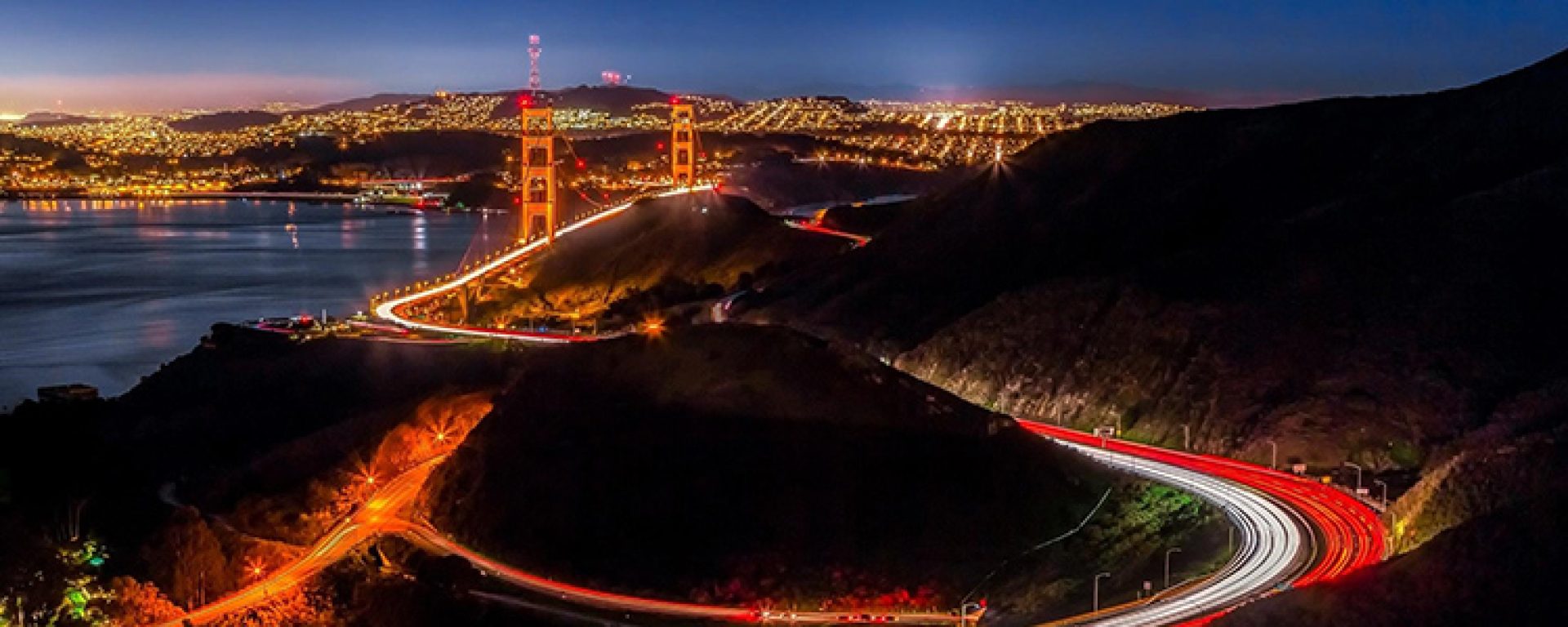
10.16 Pb/s Record!
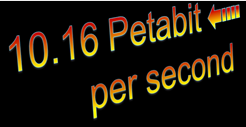
A data rate of 10 Pb/s means that 1015 bits or 1.25 million Go are exchanged every second. To give you an idea of what this magnitude entails, the entire population of Europe could watch Netflix at the same time with a connection of 10 Pb/s. 10 Pb/s is actually the world record for transmission capacity through a single optical fiber. It was obtained by the Japanese telecom operator KDDI and Sumitomo Electric Industries (SEI) in 2017, with the help of Cailabs.
Even if you have fibers at home, you definitely don’t have a millionth of this connection. So how was this technical feat accomplished? What did KDDI do differently with the fiber and what elements made it possible to achieve such a high transmission capacity?
Internet and global traffic
This is now a well-known fact: the amount of data exchanged follows a vertiginous growth pattern. In addition to what’s known as “fixed” Internet, new uses such as the widespread use of mobile Internet, the Internet of Things and 5G tend to increase connection requirements, to the point that traffic is expected to double every two to three years. The optical fiber network, which constitutes the physical channel for the exchange of the Internet, could therefore become saturated.
Fortunately, strategies are being studied to increase fiber transmission capacity. This is particularly the case for spatial multiplexing.
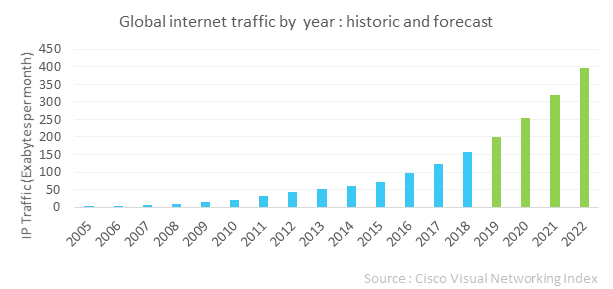
Optical fibers: the highway of light
KDDI is a Japanese telecom operator with unique expertise in fiber optic data transmission. In order to spread as much information as possible through each physical channel, the Japanese research team has managed to combine all the latest advances in fiber communications and dramatically increase fiber transmission capacity.
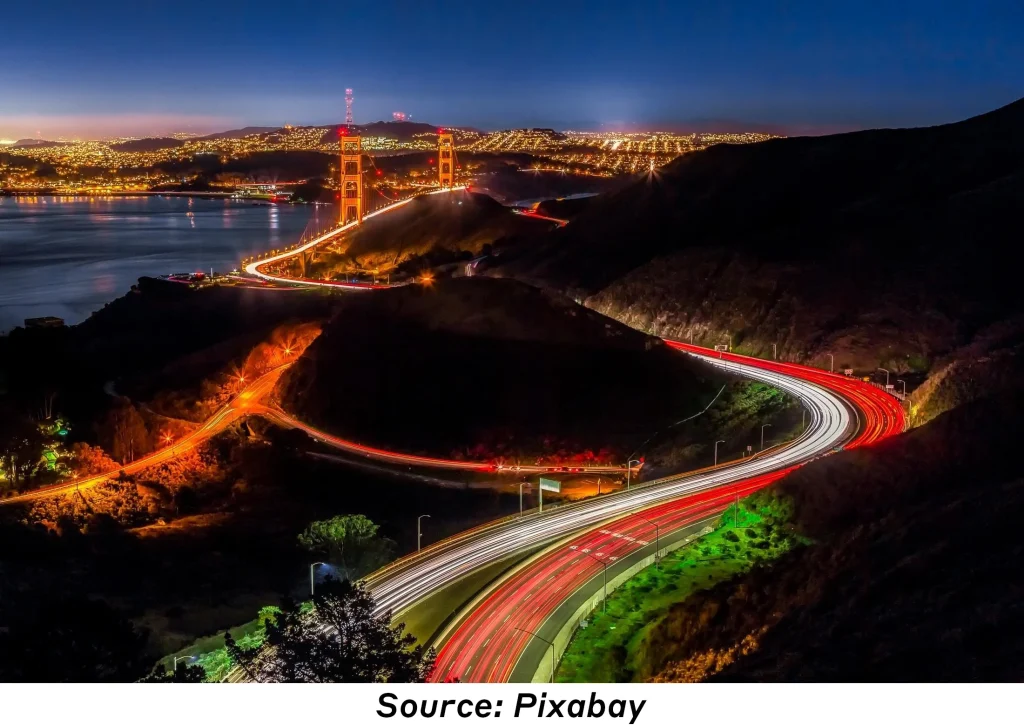
In telecommunications, we can say that transmission capacity is governed by three factors. In keeping up with the highway analogy, these factors are:
- The number of channels. It can be said that this is the number of lanes of the highway. A standard optical fiber, also known as a single-mode fiber, has a single channel.
- The coding of the information or modulation scheme, or in other words the alphabet we have available. This can be seen as the number of places in one’s car. Just as a larger car can hold more passengers, the larger the alphabet, the more information a symbol will contain. Binary code has 2 symbols, the French alphabet 26, a complex modulation scheme of the light can have up to several dozen.
- Propagation speed. This is the speed at which information is exchanged. For instance, a postman carrying a letter can at best go 50 km/h in the city. However, in optics, we are already at the maximum speed allowed: that of light. That means this is not a parameter we can play with.
All that being said, one of the objectives of optical telecommunications is to have a link with the maximum number of channels and an alphabet that’s as varied as possible.
Modulation: increasing the size of the alphabet
Increasing the alphabet in optics equates to coding information using one or more properties of light. The more varied the alphabet, the more information a symbol contains and the faster the transmission. A technique that’s commonly used with high-speed transmissions is to use amplitude and phase modulations to encode symbols. In the experiment carried out by KDDI, they used QAM modulation for quadrature amplitude modulation. This technic, as shown on the diagram below, enables for instance, that up to 64 different symbols to be coded with a 64-QAM modulation scheme. Although complex, this technology is currently known and controlled by telecom researchers.
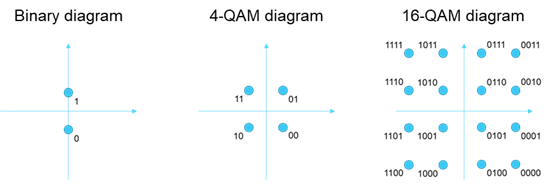
In this particular case, QAM modulation makes it possible to significantly increase the coding capacity, or modulation, of information. The next step is to increase the number of channels, that is, parallel lanes. However, the goal is still to use a single fiber. How then, can the number of lanes be increased?
Adding channels through multiplexing
To answer that question, we must take a look at multiplexing.
Multiplexing consists of creating parallel, independent information channels using specific light properties. Let’s take wavelength, or the color of the light, as an example. If we consider two sufficiently spaced wavelengths, they can propagate independently in the same optical fiber. Unfortunately, this is not the case of a red and blue car going down the same highway. However, in optics, energy is not exchanged between two wavelengths. We could say that they do not cross, as though on different floors. It is therefore possible to encode information on each of them and in doing so, to double the transmission capacity. In practice, optical fibers can accept a very large number of wavelengths, up to several hundred, increasing transmission capacity accordingly.
This technology has largely contributed to the increase in traffic in optical fibers. In 2012, the use of several hundred wavelengths enabled the KDDI research team to achieve a rate of 0.1 Pb/s in a single single-mode fiber! In continuing with the Netflix comparison, 0.1 Pb/s equates to 5 million users streaming at the time. Unfortunately, with these rates, we are approaching the limits of standard single-mode fibers and wavelength-division multiplexing. Other solutions are needed to increase the number of channels. This is where Cailabs and spatial multiplexing come in.
Spatial multiplexing: multi-core vs. multi-mode, Kezako?
In order to further increase the number of channels, spatial multiplexing is indeed necessary. The principle behind spatial multiplexing is to directly use several spatial channels at the same time. But what does that mean exactly?
Traditionally, it can be implemented in two variations depending on the type of fiber used. I will discuss different fiber types in a later article, but, schematically, we can say that in an optical fiber, light spreads in the core. The core is an area of reduced size in the center of the fiber, allowing light to be confined by total internal reflection.
To obtain several spatial channels, the first technique is to simply construct a fiber with several cores, also known as a multi-core fiber, as shown on the image below. There are several kinds, coupled and non-coupled, but it can be considered at its base that each channel can propagate its own information.
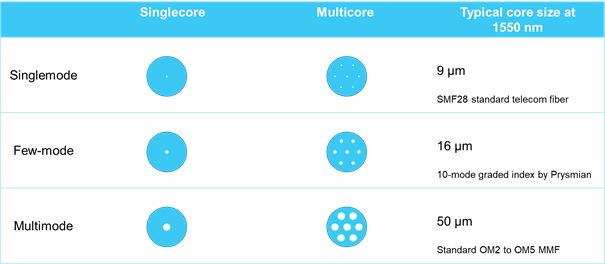
The other approach we’re developing at Cailabs is light shaping. Several light shapes can spread within a fiber whose core has a larger diameter, also known as few-mode or multi-mode fibers, depending on its size. These shapes, known as spatial modes (illustrated below), are said to be orthogonal to one another and cannot exchange energy. They propagate independently and are able to carry their own information. In the same way as wavelength-division multiplexing, the use of several spatial modes makes it possible to increase the transmission capacity by a factor equal to the number of modes.
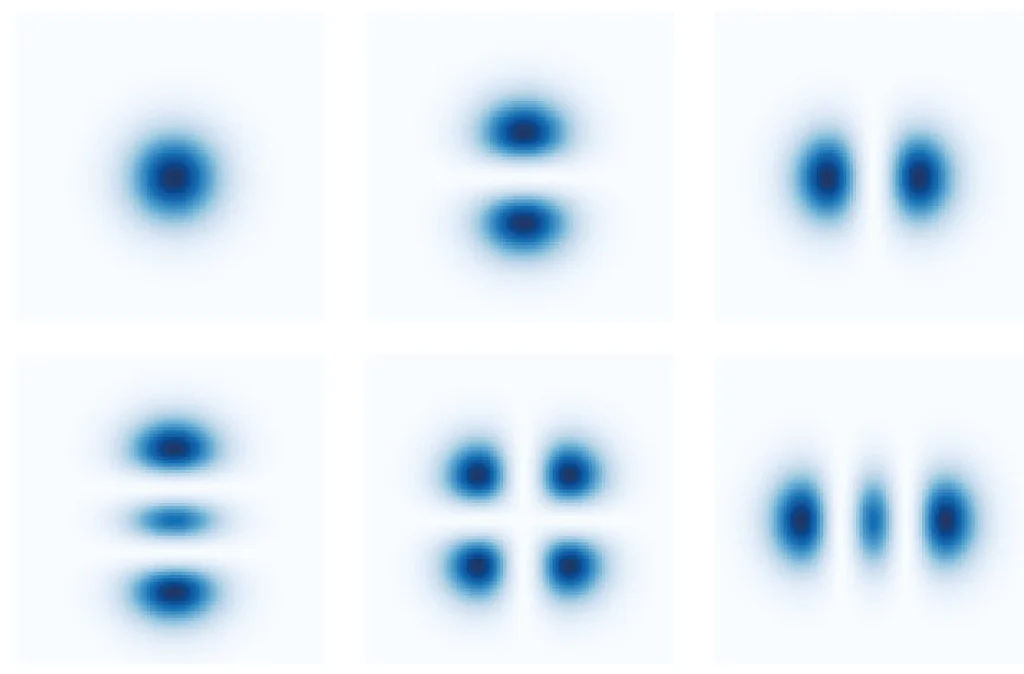
The KDDI record
The strength of KDDI researchers was in their ability to combine all of these innovations and push them to their technological limits. A single fiber, both few-mode and multi-core, of 11.3 km was specially manufactured by SEI to meet KDDI’s needs. This fiber has 19 cores, each of which can propagate 6 different spatial modes. Thus, a total of 114 spatial channels are accessible on this fiber. In each spatial mode, the researchers then multiplexed 739 wavelengths. Lastly, 16 to 64 modulation symbols were used on each wavelength.
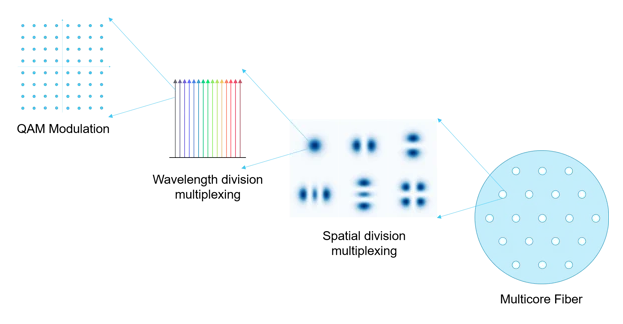
To summarize, we have:
- 16 to 64 symbols per wavelength
- 739 wavelengths per spatial mode
- 6 spatial modes per core
- 19 fiber cores
———————————
= 10.16 Pb / s
Overall, there are 114 spatial channels on which a total of 84,246 channels can be propagated with wavelength division multiplexing, all on a single optical fiber. It is truly an optical highway with over 84,000 lanes. It was therefore by drawing on the contributions of each of the latest technological innovations in modulation and multiplexing, mainly spatial, that KDDI was able to reach the tremendous speed of 10.16 Pb/s.
When will I be able to get 10.16 Pb/s at home?
Right now, these rates are still restricted to laboratory experiments. But at Cailabs, we are already working with KDDI and other researchers around the world to develop the networks of tomorrow through our PROTEUS spatial multiplexer solution. Although these technologies may not be deployed immediately for the global internet, spatial multiplexing and light shaping has proven itself as a, if not the only, solution to increasing transmission capacity in local networks, on airplanes and even for satellite communications.
So, if streaming is slow tonight and you can’t watch Netflix, let it be known that Cailabs is working on shaping today’s light to improve tomorrow’s networks.
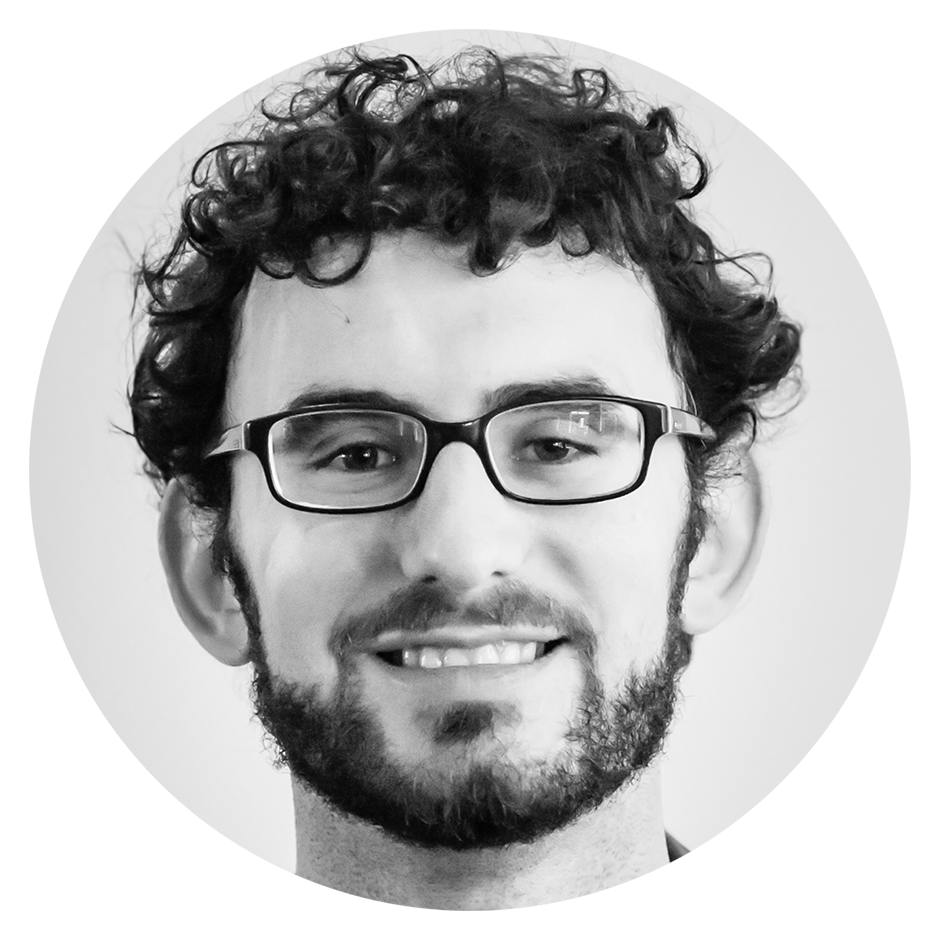
By David Allioux
David Allioux a obtenu son doctorat en physique à l’Université de Lyon. Il a rejoint Cailabs en 2018 en tant que Chef de projet et de produits. Il est responsable du développement et de l’intégration de solutions R&D en télécommunications. Il apporte également son expertise au développement et à la commercialisation de la gamme de produits dédiée aux communications laser.